What is Epigenetics?
Epigenetics is the study of heritable changes in gene expression (active versus inactive genes) that do not involve changes to the underlying DNA sequence — a change in phenotype without a change in genotype — which in turn affects how cells read the genes. Epigenetic change is a regular and natural occurrence but can also be influenced by several factors including age, the environment/lifestyle, and disease state. Epigenetic modifications can manifest as commonly as the manner in which cells terminally differentiate to end up as skin cells, liver cells, brain cells, etc. Or, epigenetic change can have more damaging effects that can result in diseases like cancer. At least three systems including DNA methylation, histone modification and non-coding RNA (ncRNA)-associated gene silencing are currently considered to initiate and sustain epigenetic change.1 New and ongoing research is continuously uncovering the role of epigenetics in a variety of human disorders and fatal diseases.
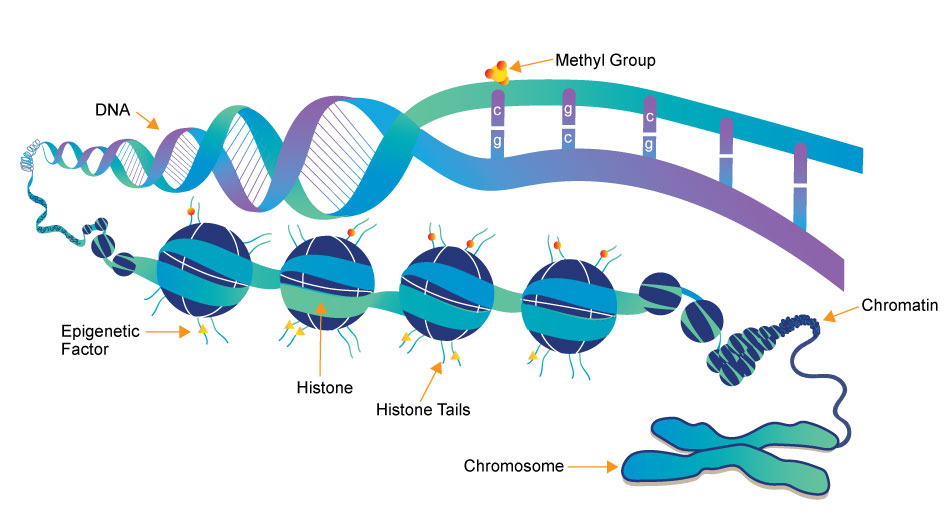
The Evolving Landscape of Epigenetic Research: A Brief History
What began as broad research focused on combining genetics and developmental biology by well-respected scientists including Conrad H. Waddington and Ernst Hadorn during the mid-twentieth century has evolved into the field we currently refer to as epigenetics. The term epigenetics, which was coined by Waddington in 1942, was derived from the Greek word “epigenesis” which originally described the influence of genetic processes on development.2 During the 1990s there became a renewed interest in genetic assimilation. This led to elucidation of the molecular basis of Conrad Waddington’s observations in which environmental stress caused genetic assimilation of certain phenotypic characteristics in Drosophila fruit flies. Since then, research efforts have been focused on unraveling the epigenetic mechanisms related to these types of changes.3
Currently, DNA methylation is one of the most broadly studied and well-characterized epigenetic modifications dating back to studies done by Griffith and Mahler in 1969 which suggested that DNA methylation may be important in long term memory function.4 Other major modifications include chromatin remodeling, histone modifications, and non-coding RNA mechanisms. The renewed interest in epigenetics has led to new findings about the relationship between epigenetic changes and a host of disorders including various cancers, mental retardation associated disorders, immune disorders, neuropsychiatric disorders and pediatric disorders.
Epigenetics and the Environment: How Lifestyle Can Influence Epigenetic Change from One Generation to the Next
The field of epigenetics is quickly growing and with it the understanding that both the environment and individual lifestyle can also directly interact with the genome to influence epigenetic change. These changes may be reflected at various stages throughout a person’s life and even in later generations. For example, human epidemiological studies have provided evidence that prenatal and early postnatal environmental factors influence the adult risk of developing various chronic diseases and behavioral disorders.5 Studies have shown that children born during the period of the Dutch famine from 1944-1945 have increased rates of coronary heart disease and obesity after maternal exposure to famine during early pregnancy compared to those not exposed to famine.6 Less DNA methylation of the insulin-like growth factor II (IGF2) gene, a well-characterized epigenetic locus, was found to be associated with this exposure.7 Likewise, adults that were prenatally exposed to famine conditions have also been reported to have significantly higher incidence of schizophrenia.89
Research has also shown that a mother’s exposure to pollution could impact her child’s asthma susceptibility and her intake of vitamin D could change DNA methylation that influences placenta functioning. It doesn’t stop at the mother, however, as further studies support that the father has a hand in his child’s health and epigenetic marks as well. Read: A Child’s Mental Fitness Could Be Epigenetically Influenced by Dad’s Diet.
How Lifestyle Can Affect Individual Epigenetics and Health
Although our epigenetic marks are more stable during adulthood, they are still thought to be dynamic and modifiable by lifestyle choices and environmental influence. It is becoming more apparent that epigenetic effects occur not just in the womb, but over the full course of a human life span, and that epigenetic changes could be reversed. There are numerous examples of epigenetics that show how different lifestyle choices and environmental exposures can alter marks on top of DNA and play a role in determining health outcomes.
The environment is being investigated as a powerful influence on epigenetic tags and disease susceptibility. Pollution has become a significant focus in this research area as scientists are finding that air pollution could alter methyl tags on DNA and increase one’s risk for neurodegenerative disease. Interestingly, B vitamins may protect against harmful epigenetic effects of pollution and may be able to combat the harmful effects that particular matter has on the body.
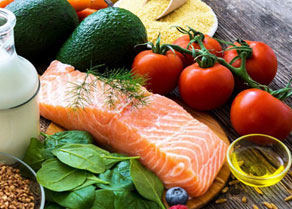
Diet has also been shown to modify epigenetic tags in significant ways. The field of nutriepigenomics explores how food and epigenetics work together to influence health and wellbeing. For example, a study found that a high fat, low carb diet could open up chromatin and improve mental ability via HDAC inhibitors. Other studies have found that certain compounds within the foods we consume could protect again cancer by adjusting methyl marks on oncogenes or tumor suppressor genes. Ultimately, an epigenetic diet may guide people toward the optimal food regimen as scientific studies reveal the underlying mechanisms and impact that different foods have on the epigenome and health.
Explore these topics in epigenetics:
![]() |
![]() |
![]() |
![]() |
![]() |
![]() |
![]() DIET |
![]() |
Clinical Applications – Epigenetic Diseases
An accumulation of genetic and epigenetic errors can transform a normal cell into an invasive or metastatic tumor cell.
Cancer. Cancer was the first human disease to be linked to epigenetics. Studies performed by Feinberg and Vogelstein in 1983, using primary human tumor tissues, found that genes of colorectal cancer cells were substantially hypomethylated compared with normal tissues.10 DNA hypomethylation can activate oncogenes and initiate chromosome instability, whereas DNA hypermethylation initiates silencing of tumor suppressor genes. An accumulation of genetic and epigenetic errors can transform a normal cell into an invasive or metastatic tumor cell. Additionally, DNA methylation patterns may cause abnormal expression of cancer-associated genes. Global histone modification patterns are also found to correlate with cancers such as prostate, breast, and pancreatic cancer. Subsequently, epigenetic changes can be used as biomarkers for the molecular diagnosis of early cancer.
Mental Retardation Disorders. Epigenetic changes are also linked to several disorders that result in intellectual disabilities such as ATR-X, Fragile X, Rett, Beckwith-Weidman (BWS), Prader-Willi and Angelman syndromes.1 For example, the imprint disorders Prader-Willi syndrome and Angelman syndrome, display an abnormal phenotype as a result of the absence of the paternal or maternal copy of a gene, respectively. In these imprint disorders, there is a genetic deletion in chromosome 15 in a majority of patients. The same gene on the corresponding chromosome cannot compensate for the deletion because it has been turned off by methylation, an epigenetic modification. Genetic deletions inherited from the father result in Prader-Willi syndrome, and those inherited from the mother, Angelman syndrome.
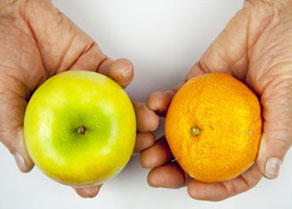
Immunity & Related Disorders. There are several pieces of evidence showing that loss of epigenetic control over complex immune processes contributes to autoimmune disease. Abnormal DNA methylation has been observed in patients with lupus whose T cells exhibit decreased DNA methyltransferase activity and hypomethylated DNA. Disregulation of this pathway apparently leads to overexpression of methylation-sensitive genes such as the leukocyte function-associated factor (LFA1), which causes lupus-like autoimmunity. Interestingly, LFA1 expression is also required for the development of arthritis, which raises the possibility that altered DNA methylation patterns may contribute to other diseases displaying idiopathic autoimmunity. Epigenetic research has also shown that there is joint-specific DNA methylation and transcriptome signatures in rheumatoid arthritis, which could help explain why some targeted therapies for arthritis could alleviate pain in the knees but not the hips.
Neuropsychiatric Disorders. Epigenetic errors also play a role in the causation of complex adult psychiatric, autistic, and neurodegenerative disorders. Several reports have associated schizophrenia and mood disorders with DNA rearrangements that include the DNMT genes. DNMT1 is selectively overexpressed in gamma-aminobutyric acid (GABA)-ergic interneurons of schizophrenic brains, whereas hypermethylation has been shown to repress expression of Reelin (a protein required for normal neurotransmission, memory formation and synaptic plasticity) in brain tissue from patients with schizophrenia and patients with bipolar illness and psychosis. A role for aberrant methylation mediated by folate levels has been suggested as a factor in Alzheimer’s disease; also some preliminary evidence supports a model that incorporates both genetic and epigenetic contributions in the causation of autism. Autism has been linked to the region on chromosome 15 that is responsible for Prader-Willi syndrome and Angelman syndrome. Findings at autopsy of brain tissue from patients with autism have revealed a deficiency in MECP2 expression that appears to account for reduced expression of several relevant genes.
Pediatric Syndromes. In addition to epigenetic alterations, specific mutations affecting components of the epigenetic pathway have been identified that are responsible for several syndromes: DNMT3B in ICF (immunodeficiency, centromeric instability and facial anomalies) syndrome, MECP2 in Rett syndrome, ATRX in ATR-X syndrome (a-thalassemia/mental retardation syndrome, X-linked), and DNA repeats in facioscapulohumeral muscular dystrophy. In Rett syndrome, for example, MECP2 encodes a protein that binds to methylated DNA; mutations in this protein cause abnormal gene expression patterns within the first year of life. Girls with Rett syndrome display reduced brain growth, loss of developmental milestones and profound mental disabilities. Similarly, the ATR-X syndrome also includes severe developmental deficiencies due to loss of ATRX, a protein involved in maintaining the condensed, inactive state of DNA. Together, this constellation of clinical pediatric syndromes is associated with alterations in genes and chromosomal regions necessary for proper neurologic and physical development.
The increased knowledge of epigenetics, combined with rise of technologies such as CRISPR/Cas9 gene editing and next-generation sequencing in recent years, allows us to better understand the interplay between epigenetic change, gene regulation, and human diseases, and will lead to the development of new approaches for molecular diagnosis and targeted treatments across the clinical spectrum.
Ready to learn about the first epigenetic mechanism? Read on: DNA Methylation