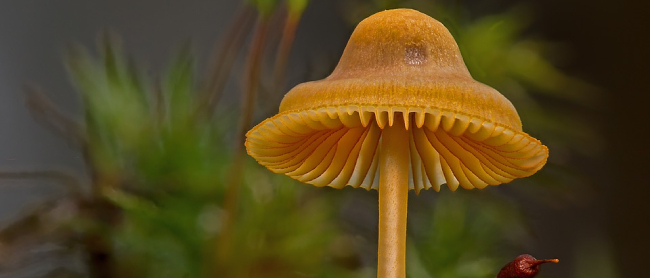
Decades of antibiotic and antifungal use have dramatically impacted the global microbiome, with ramifications for agriculture and human health. Worries are mounting but no systematic plans of action are forthcoming. Though that is not to say there are no slivers of hope.
While they are still largely confined to immunocompromised patients, fungal infections are a looming public health crisis. Invasive candidiasis is increasingly caused by multidrug-resistant non-albicans yeasts and nearly 60% of C. parapsilosi sampled in South Africa are now fluconazole-resistant, alarmingly but not abnormally high (Friedman, 2019).
For agriculture fungal pathogens pose a more immediate threat. Highly resistant species like F. oxysporum, responsible for Panama disease in bananas, could ruin the livelihoods of millions of farmers (Ordonez, 2015).
Rising global temperatures will only hasten the emergence of new threats. Catastrophic events brought on by fungus are not unknown. Between 1845 and 1852, P. infestans led to a million deaths and the emigration of 2.1 million Irish.
The decimation of a staple or cash crop is becoming more probable as mycologists are not only finding newly resistant pathogens but newly pathogenic species (Nnadi, 2021; Fischer 2012).
Yet for now there are only four classes of antifungals in common use: azoles, polyenes, echinocandins, and a single nucleoside analog, flucytosine (McCarthy, 2019).
The documented genetic changes in bacterial populations do not fully explain antibiotic resistance, which has prompted researchers to turn their attention to epigenetic processes (Ghosh, 2020). Many of these alterations have been studied in oncological contexts, culminating in multiple FDA-approve dcancer therapeutics (Heerboth et. al, 2014).
Common epigenetic modifications include acetylation, methylation, sumoylation, alterations to chromatin structure, and, perhaps most promising for the management of infectious diseases, RNA silencing.
These mechanisms, alone or together, foster phenotypic plasticity. Rapid epigenetic responses allow unwanted cells (malignant, bacterial, or fungal) to buy time while heritable adaptations are evolved.
Histone deacetylases (HDACs) remove acetyl groups from histones, which are partially responsible for managing cellular stress responses. Inhibiting HDACs impacts virulence factors like biofilm formation, fungal growth, and host dissemination (Garnaud, 2016; Simonetti, 2007).
Histone acetylation is involved in the development of antifungal resistance in C. albicans; it’s been known for decades that Trichostatin A, a broad-spectrum HDAC inhibitor (HDACi), has antifungal properties; as coordinators of fungal life cycles, targeting histones can reduce virulence, as was shown with U. maydis, better known as Corn Smut (Elías-Villalobos, 2015).
Other epigenetic phenomena, like RNA silencing, prominently figure into how pathogenic fungi operate and how, in the near future, they can be managed.
RNAi signals can affect the cells of more than one organism. Pathogens utilize effector proteins and toxins to suppress innate host immunity. It was thought fungi’s toolkit was limited to these factors until Weinberg found that B. cinerea, better known as Soft Rot, produces sRNAs to disrupt immune signaling pathways in plants (Hua, 2018).
In other words, they can be used by a host to defend or a parasite to attack (or vice versa). This line of inquiry was opened in 1998 when Timmons and Fire fed dsRNA-bearing E. coli to nematodes. Host-induced gene silencing (HIGS), under human control, was born.
It has been applied to Wilt Disease in arabidopsis and cotton. Wilt can kill a tree within a single growing season. It is doubly concerning because no resistance genes have been found in crops like cotton, sunflowers, and potatoes (Zhang, 2016).
RNA silencing in M. circinelloides (which does not yet have a more memorable common name) gives us an example of the previously mentioned phenomenon of phenotypic plasticity.
M. circinelloides gains 5-FOA resistance through sense and antisense sRNAs, which silence pyRf and pyRg. This is normally accomplished through genetic mutations in these loci. A similar alteration simultaneously protects it from rapamycin and FK506 (Calo et. al, 2014).
It is vital not only to consider new techniques, but how to enhance the efficacy of existing options. This can be done through epigenetic modulation (Chang et. al, 2019), which has been explored with antibiotic resistant bacteria. It offers an expedient manner of prolonging the usefulness of approved antifungals (Adam, 2008; Ghosh, 2020).
Despite the costs of remaining unprepared, relevant diagnostic tests, vaccines, drugs, and basic pathophysiological research remains lamentably underfunded (Brown et. al, 2012). Moreover, a dangerous overlap between antifungals used for plants and humans is driving drug resistance, as attested to animal pathogens repeatedly acquiring azole resistance through agricultural fungicides (Berger, 2017).
If these avenues are not pursued now, we will find ourselves caught off guard yet again. Unpreparedness is not tenable in a hyperconnected world.
References
Adam, M., Murali, B., Glenn, N.O. et al. (2008). Epigenetic inheritance based evolution of antibiotic resistance in bacteria. BMC Evol Biol 8, 52.
Berger, S., El Chazli, Y., Babu, A. F., & Coste, A. T. (2017). Azole Resistance in Aspergillus fumigatus: A Consequence of Antifungal Use in Agriculture? Frontiers in microbiology, 8, 1024.
Billmyre, R. B., Calo, S., Feretzaki, M., Wang, X., & Heitman, J. (2013). RNAi function, diversity, and loss in the fungal kingdom. Chromosome Res. 21, 561–72.
Brown, G. D., Denning, D. W., Gow, N. A., Levitz, S. M., Netea, M. G., & White, T. C. (2012). Hidden killers: human fungal infections. Sci Transl Med. 4(165)
Chang Z, Yadav V, Lee SC, Heitman J. (2019) Epigenetic mechanisms of drug resistance in fungi. Fungal Genet Biol.
Chang, Z., Heitman J. (2019). “Drug-resistant epimutants exhibit organ-specific stability and induction during murine infections caused by the human fungal pathogen Mucor circinelloides.” MBio 10.6
Elías-Villalobos, Alberto, et al. “The Hos2 histone deacetylase controls Ustilago maydis virulence through direct regulation of mating-type genes.” PLoS pathogens 11.8 (2015): e1005134.
Fisher MC, Henk DA, Briggs CJ, et al. (2012). Emerging fungal threats to animal, plant and ecosystem health. Nature. 484.186-194.
Friedman DZP, Schwartz IS. (2019). Emerging Fungal Infections: New Patients, New Patterns, and New Pathogens. J Fungi (Basel). 2019;5(3):67.
Garnaud C, Champleboux M, Maubon D, Cornet M, Govin J. (2016). Histone Deacetylases and Their Inhibition in Candida Species. Front Microbiol. 7(1238).
Ghosh D, Veeraraghavan B, Elangovan R, Vivekanandan P. (2020). Antibiotic Resistance and Epigenetics: More to It than Meets the Eye. Antimicrob Agents Chemother. 64(2).
Halsall, John A., and Bryan M. Turner. (2016). “Histone deacetylase inhibitors for cancer therapy: An evolutionarily ancient resistance response may explain their limited success.” Bioessays. 38.(11).102-1110.
Heerboth, Sarah, et al. (2014). “Use of epigenetic drugs in disease: an overview.” Genetics & Epigenetics. 6. 9-19.
Hua, Chenlei, Jian-Hua Zhao, and Hui-Shan Guo. (2018) “Trans-kingdom RNA silencing in plant–fungal pathogen interactions.” Molecular Plant 11(2) 235-244.
Nnadi NE, Carter DA. Climate change and the emergence of fungal pathogens. PLoS Pathog.17(4)
Ordonez N, Seidl MF, Waalwijk C, Drenth A, Kilian A, Thomma BP, Ploetz RC, Kema GH. (2015). Worse Comes to Worst: Bananas and Panama Disease–When Plant and Pathogen Clones Meet. PLoS Pathog. 11(11)
Simonetti, Giovanna, et al. (2007) “Histone deacetylase inhibitors may reduce pathogenicity and virulence in Candida albicans.” FEMS yeast research 7.8 (2007): 1371-1380.
Sinha S, Tyagi C, Goyal S, Jamal S, Somvanshi P, Grover A. (2016). Fragment based G-QSAR and molecular dynamics based mechanistic simulations into hydroxamic-based HDAC inhibitors against spinocerebellar ataxia. J Biomol Struct Dyn. 34(10):2281-95.
Timmons, L., & Fire, A. (1998). Specific interference by ingested dsRNA. Nature, 395(6705), 854.
Torres-Martinez S, Ruiz-Vazquez RM, (2016). RNAi pathways in Mucor: A tale of proteins, small RNAs and functional diversity. Fungal Genet Biol. 90, 44–52.
Zhang T, Jin Y, Zhao JH, Gao F, Zhou BJ, Fang YY, Guo HS. (2016). Host-Induced Gene Silencing of the Target Gene in Fungal Cells Confers Effective Resistance to the Cotton Wilt Disease Pathogen Verticillium dahliae. Mol Plant. 9(6):939-42.