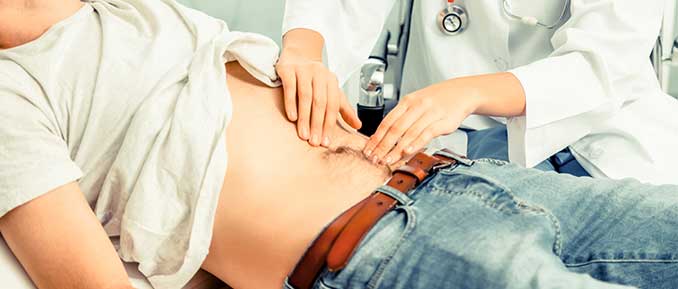
The barrier of the small intestine consists of a thin layer of cells which blocks pathogens from entering the body and has a major role in absorbing nutrients through microvilli. Maintenance of the intestinal barrier is important since these cells are constantly being sloughed off as food passes through and tiny amounts of damage can cause a leaky gut which is now understood to be associated with several chronic diseases (Fasano 2017 and Meddings 2006).
The intestinal barrier is normally completely shed and replaced over the course of only one week and astonishingly is only a single cell thick. One enzyme that helps direct cell proliferation and tissue repair of the gut barrier is histone deacetylase 3 (HDAC3). HDAC3 is an epigenetic regulator of gene expression and prevents transcription of certain genes when activated. Therefore, HDAC3 plays an important role in maintenance of the intestinal barrier which is constantly being renewed.
The gut microbiome adds an additional layer of protection by secreting a layer of mucus and producing molecules which regulate HDAC3 activity. Some microbes produce a short-chain fatty acid called butyrate, which is a signaling molecule known to inhibit HDAC enzymes. However, microbes also produce inositol triphosphate (IP3) which activates HDAC enzymes.
Researchers at Cincinnati Children’s Hospital Medical Center recently found that mice with a normal microbiome which produces butyrate have paradoxically increased HDAC activity, particularly of HDAC3. To investigate why this might be, the authors housed mice in either a conventional (Conv) or germ-free environment which prevents microbial colonization of the gut. By conducting this experiment, the authors were able to determine the role of the gut microbiome in modulating HDAC activity.
Intestinal cells from Conv mice showed elevated IP3 while cells isolated from the germ-free mice did not. The authors proposed that IP3 signaling may be the major factor stimulating HDAC3 activity. IP3 is produced by microbial conversion of phosphate in the human diet, which is often present as phytate in many plant-based foods such as wheat and oats. Phytate requires processing by microbial phytase before it can be absorbed and utilized.
Importantly, adding phytate to the diets of germ-free mice in this study did not increase HDAC3 activity indicating that the microbiome plays an important role in processing phytate and providing IP3 to the epithelial cells and therefore activating HDAC3 signaling.
The authors next wanted to determine if colonizing mice with bacteria that either were or were not able to break down phytate would affect HDAC3 activity. Mice colonized with bacteria unable to break down phytate showed less available IP3 and reduced HDAC3 activation.
The authors wanted to observe the significance of these results in a model of intestinal damage since HDAC3 is an important modulator of cell proliferation and repair. The authors treated mice with dextran sodium sulfate (DSS) which causes extensive damage to the gut similar to that seen in humans with ulcerative colitis. Germ-free mice were more sensitive to DSS-induced damage and died quicker than Conv mice.
However, rectally administered IP3 was able to somewhat improve survivability of germ-free mice. The authors repeated this experiment but used antibiotic-treated mice instead of germ-free mice to observe whether the beneficial effect of IP3 treatment would hold true in a less severe model of an impaired microbiome. IP3 treatment improved survivability of antibiotic-treated mice up to a level similar to the Conv mice.
Since DSS-induced damage resembles damage seen in ulcerative colitis, data from the Human Microbiome Project Cohort was examined to determine if microbiome samples from ulcerative colitis patients have a difference in phytase abundance. Indeed, phytase was less abundantly expressed by in ulcerative colitis patients.
The authors then used phytate as a rescue treatment in their DSS-treated mice. Phytate did not affect survival of germ-free mice administered DSS but sharply improved survival in Conv mice. Importantly, this means as long as the microbiome was intact, the treatment had a positive effect. These mice also showed less infiltration of T-cells to the gut which indicates less severe inflammation.
These results show the microbiome plays an important role in breaking down dietary phytate that is able to activate IP3/HDAC3 signaling and stimulate intestinal tissue repair. The authors bolstered this data by performing additional experiments in an intestinal organoid model which is a tiny lab-grown piece of tissue that mimics the human gut. They found treating intestinal organoids with IP3 stimulated the proliferation of epithelial cells and promoted tissue growth.
In conclusion, the authors described the exciting new finding that IP3 generated by bacterial conversion of dietary phytate could activate HDAC3 and promote proliferation and repair of the intestinal epithelium after being challenged with DSS. Importantly, the gut can sense microbial products which direct repair of the host intestinal barrier. This study improves our basic understanding of the importance of host-microbe interactions in intestinal biology by epigenetic mechanisms.
While phosphate is abundant in the human diet and an unlikely nutrient deficiency, this study shows a potential therapeutic role of phosphate during intestinal damage. Alternatively, precision probiotics which express phytate could be investigated for potential of improving tissue repair. The concept of using probiotics and similarly, fecal transplants, for treating certain gut conditions and now even obesity is rapidly gaining popularity (Brandt 2012 and Zhang 2019).
Source:
Wu S., Hashimoto-Hill S., et al. (2020). Microbiota-derived metabolite promotes HDAC3 activity in the gut. Nature.
References:
Fasano A. (2017). Gut permeability, obesity, and metabolic disorders: who is the chicken and who is the egg?. Am J Clin Nutr. 2017; 105(1):3-4.
Meddings J. (2008). The significance of the gut barrier in disease. Gut. 57(4):438-440.
Brandt LJ. (2012). Fecal transplantation for the treatment of Clostridium difficile infection. Gastroenterol Hepatol (N Y). 8(3):191-194.
Zhang Z, Mocanu V, Cai C, et al. (2019). Impact of Fecal Microbiota Transplantation on Obesity and Metabolic Syndrome-A Systematic Review. Nutrients. 11(10):2291.